Essential chemistry for biochemists - PubMed
Amanda L Jonsson 1 ,
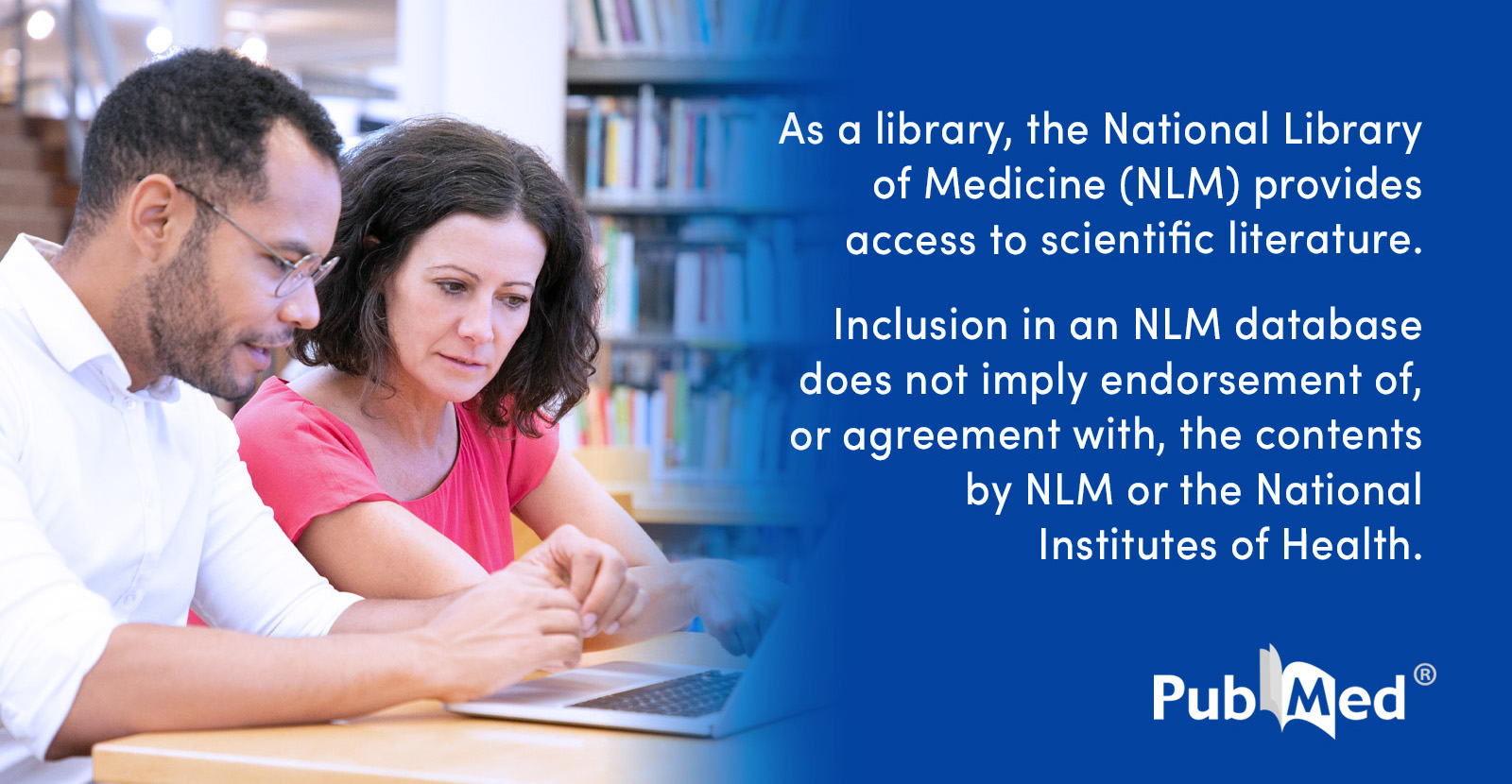
Review
. 2017 Oct 31;61(4):401-427.
doi: 10.1042/EBC20160094. Print 2017 Oct 31.
Affiliations
- PMID: 28951470
- PMCID: PMC5869253
- DOI: 10.1042/EBC20160094
Review
Essential chemistry for biochemists
Amanda L Jonsson et al. Essays Biochem. .
Abstract
Within every living organism, countless reactions occur every second. These reactions typically occur more rapidly and with greater efficiency than would be possible under the same conditions in the chemical laboratory, and while using only the subset of elements that are readily available in nature. Despite these apparent differences between life and the laboratory, biological reactions are governed by the same rules as any other chemical reaction. Thus, a firm understanding of the fundamentals of chemistry is invaluable in biochemistry. There are entire textbooks devoted to the application of chemical principles in biological systems and so it is not possible to cover all of the relevant topics in depth in this short article. The aim is instead to provide a brief overview of those areas in chemistry that are most relevant to biochemistry. We summarize the basic principles, give examples of how these principles are applied in biological systems and suggest further reading on individual topics.
Keywords: Chemical reactions; Functional groups; Non covalent interactions.
© 2017 The Author(s).
Conflict of interest statement
The authors declare that there are no competing interests associated with the manuscript.
Figures

The 28 elements essential for animal life are indicated by coloured squares; trace elements are shown in yellow and those present in larger quantities are shown in green. The six most abundant elements in the human body are carbon, hydrogen, oxygen, nitrogen, phosphorus and calcium, accounting for almost 99% of the mass of an adult human. Carbon, hydrogen, oxygen and nitrogen are the building blocks of organic biomolecules, calcium is present in large amounts in bones and teeth (in addition to being vital for cell signalling in smaller amounts), phosphorus is likewise found in bones and teeth (smaller quantities are a vital part of DNA, adenoside triphosphate – the energy currency of the cell – and play an important role in cell signalling).

In these images, atomic orbitals and bonds are depicted as line drawings indicating shape and as isosurfaces, regions of space enclosing a defined fraction of the electron density. (A) When two s-orbitals overlap, a σ-bond is formed. In σ bonds, the atomic orbitals overlap ‘head on’ and there is electron density between the two nuclei in the bond. As a result, these bonds are typically strong compared with π bonds between the same elements. (B) In a π bond, two p-orbitals overlap laterally, and the nuclei lie in a plane in which there is no electron density. There is less overlapping between orbitals in a π bond than in a σ bond and so these bonds are typically weaker. It should be noted that a double bond, consisting of a σ and π bond is stronger than a single bond between the same two elements as the strength of both the σ and π components are included in the bond strength. (C) When a bond is formed between carbon and oxygen, there will be more electron density located near the oxygen atom, as illustrated here in the isosurface for a carbon–oxygen π bond.

(A) Each of the six carbon atoms in benzene contribute a p-orbital to the delocalized system. A circle in the centre of the ring can be used to highlight the fact that the system is delocalized, however many biochemists prefer to use the alternating bond representation. (B) The four bases found in DNA, all have delocalized rings of p-orbitals; in this diagram atoms shown in red each contribute a p-orbital to the delocalized system. It is not immediately obvious that the atoms indicated with an arrow can contribute a p-orbital, however using a more sophisticated approach to bonding we can show that this is the case. Adenine and guanine each have ten electrons in a delocalized ring, while cytosine and thymine have six. (C) Representation of the delocalized carboxylate anion. In this system, four electrons two from the double bond and two from the negatively charged oxygen are delocalized over three atoms. (D) Retinal has a linear delocalized system including 12 p-orbitals. Each of the atoms that contributes a p-orbital to the delocalized system is shown in red.

Water molecules form an ordered structure around a hydrophobic molecule (shown in grey). When two hydrophobic molecules aggregate the surface area exposed to water is reduced; this reduces the number of ordered water molecules in the hydration shell. Having more water molecules disordered in solution is entropically favourable.

The skeletal representation makes use of the fact that carbon and hydrogen are the most common elements in organic compounds, and also that carbon almost always forms four covalent bonds. (A) The process of converting a displayed formula into a skeletal formula has three stages: (i) the molecule is drawn with the carbon chain ‘staggered’ in a zigzag; this is important to allow correct identification of the number of carbon atoms in the final molecule and is a better representation of the actual bond angles. (ii) The ‘C’ label for carbon atoms is omitted. (iii) The ‘H’ label for hydrogen atoms is omitted only for hydrogen atoms bonded to carbon. When interpreting a skeletal formula, we assume that carbon will make four bonds; if a carbon appears to make fewer than four bonds in the skeletal representation, then the ‘missing’ bonds are assumed to be to hydrogen atoms. Using the skeletal representation highlights the heteroatoms present in the molecule and becomes particularly useful once we consider how to display different isomers. (B) The guidelines for drawing skeletal structures are relatively flexible and in some cases, for example where we wish to draw attention to a particular group of atoms, the ‘C’ symbol for some of the carbon atoms may be shown. It is important not to omit any hydrogen atoms bonded to carbon atoms that are explicitly displayed in this way. (C) Wedge and hashed bonds can be used to illustrate the direction of bond vectors relative to the plane of the page.

(A) This figure illustrates the some of the functional groups that occur frequently in biological systems. In these structures, the wavy bonds represent connections to the rest of the molecule. *In both amine and amide, one or both of the hydrogen atoms bonded to the nitrogen may be replaced by a carbon atom. (B) Many biological molecules include a large number of different functional groups, as illustrated by the structure of coenzyme A with the functional groups labelled in blue and red.

In this figure, the symbol R is used to denote the different functional groups that distinguish the amino acids. The amino acids that polymerize to form a polypeptide or protein are joined together through amide bonds. This bond is often referred to as a peptide bond. The carbon, oxygen and nitrogen atoms of the amide group all lie in the same plane with the lone pair on the nitrogen forming a delocalized system with the electrons from the carbon–oxygen double bond, as was described for the carboxylate anion. The planarity of this bond has important implications for protein structure.

The twenty amino acids commonly found in proteins. Functional groups not illustrated elsewhere are labelled in red. Full names and one-letter codes are given. Amino acids are categorized as non-polar, uncharged polar, acidic and basic according to the composition of their side chains. Methionine and tryptophan are classified as non-polar, despite including the polar atoms sulphur and nitrogen respectively, as both are typically found in the interior of a protein. The sulphur atom in methionine does not participate in hydrogen bonds or act as a nucleophile in enzymatic reactions. In tryptophan, the nitrogen lone pair is delocalized through the indole ring, ensuring that it is likewise unavailable as a nucleophile. Side chains which frequently form hydrogen bonds are classified as polar, those that lose a proton at physiological pH are acidic, and those that gain a proton are basic. Histidine could be considered both polar uncharged and basic as it exists in both neutral and positively charged state at physiological pH.

(A) This figure illustrates the three classes of structural isomers: chain isomers, position isomers and functional group isomers. (B) The fatty acid oleic acid has a cis-double bond and is often incorporated into the lipids forming the cell membrane. In contrast, elaidic acid which has a trans-double bond is not found in cell membranes. (C) 11-cis-retinal is converted into all-trans-retinal on absorption of light of the appropriate energy in the first step of light detection in the eye.

(A) Illustration of the application of the Cahn–Ingold–Prelog rules for describing the configuration of chiral centres. In the Cahn–Ingold–Prelog system, the four substituents around the asymmetric carbon atom are assigned a priority based on three rules: (i) consider the first atom in each substituent, highest priority is given to the atom with the highest atomic number; in case of isotopes, a higher atomic mass gives a higher priority. (ii) If two substituents have the same first atom, move away from the asymmetric carbon to the next bonded atom until a difference is reached. (iii) If the substituent contains a double or triple bond, then the atom farthest from the asymmetric carbon counts two or three times respectively. The molecule is then drawn with the lowest priority substituent at the back (into the page). If the priority of the other three substituents increases clockwise, the centre is assigned ‘R’ stereochemistry, otherwise it is ‘S”. (B) All natural proteinogenic amino acids are the
-form under the Fischer rules, when using the Cahn–Ingold–Prelog rules the natural form of cysteine is the R isomer, this is in contrast with the other amino acids, exemplified by serine, which is the S isomer.

(A) The drugs ibuprofen and thalidomide both exist as R and S isomers. In each case, the R and S isomers can be interconverted in the body and have different biological functions. (B) Illustration of the different representations of the glucose molecule. The linear form of glucose can be represented by a skeletal structure or a Fischer projection; the cyclic form, by a skeletal structure or a Haworth projection.

A hypothetical energy profile for the reaction of a substrate (S) forming product (P) in the absence of catalysis is shown in black, while the hypothetical profile for the same reaction in an enzyme (E) catalysed reaction is shown in grey. In the absence of an enzyme, there is a large activation energy (Eauncat). In the enzyme-catalysed reaction the enzyme forms a complex with the substrate (ES) and the product (EP) and lowers the energy of the highest energy species on the reaction pathway, resulting in a lower activation energy (Eaenz).

(A) A schematic titration curve for anserine, with (B) an illustration of the species present in the reaction mixture during the titration. Anserine has three groups that can exist in both protonated and unprotonated forms: a carboxylic acid, an imidazole nitrogen and an amine. At the beginning of the titration, all the three groups are protonated and the predominant species in the reaction mix is (1). As base is added, the pH approaches the pKa for the first ionizable group, the carboxylic acid, and species (2) begins to form resulting in a plateau in the titration curve. Once the majority of molecules are in form (2), the pH will begin to rise again, before the second plateau occurs due to the formation of species (3). It is the equilibrium between species (2) and (3) that is relevant at biological pH. The final plateau occurs when the fully deprotonated species (4) is formed.

(A) Illustration of a nucleophilic substitution with a negatively charged nucleophile and a leaving group. It should be noted that the nucleophile may be a neutral molecule with a lone pair of electrons and that the leaving group may also be neutral (as seen in (C)). (B) Common leaving groups in biological chemistry. (C) Many biological methylation reactions take place via a nucleophilic attack on S-adenosyl methionine. In this diagram, the leaving group is shown in blue and the nucleophile in red.

(A) Nucleophilic attack on a carbonyl group results in a species with four substituents around a central carbon atom (a tetrahedral species). Whether or not this species reacts further depends upon the nature of the X and Y groups and the nucleophile. (B) If X and Y are not good leaving groups and the nucleophile is also a poor leaving group, then the tetrahedral species will be the final product.

(A) If X and Y are not good leaving groups but the nucleophile is a good leaving group, then the reaction may reverse, as seen in the acid-catalysed reaction of a ketone with an alcohol. This reaction also occurs with aldehydes and the tetrahedral product is called a hemiacetal. (B) Formation of hemiacetal occurs during the cyclization of glucose.

(A) If the carbonyl subjected to a nucleophilic attack has a good leaving group, then nucleophilic substitution is possible. The substitution reaction proceeds via an obligatory tetrahedral species. (B) Three nucleophilic substitution reactions are important in the formation of an amide bond between two amino acids. The first reaction involves nucleophilic substitution at a phosphate group and the second nucleophilic reactions involve substitution at a carbonyl. In the first reaction, the carboxylate group attacks a phosphate of ATP releasing pyrophosphate – two phosphate groups connected together via a bridging oxygen atom – and attaching AMP to the carboxylate group. This step is energetically favourable and converts one of the oxygen atoms of the carboxylate group of the amino acid into a good leaving group. In the second reaction, the amino acid is loaded on to the tRNA ready for protein formation. In the third reaction, the enzyme ensures that the amino group of the attacking amino acid is not protonated, and this group then carries out a nucleophilic attack on another tRNA–conjugated amino acid.

(A) Illustration of a ketone and its enol form, and the mechanism of this reaction in the presence of acid and base. For the majority of species, the equilibrium lies towards the ketone rather than the enol. (B) The tautomerization of glucose gives an ene–diol, because there is an alcohol group adjacent to the carbonyl (diol, two alcohols). If, in the reverse reaction the carbonyl group is formed at the alcohol adjacent to the original carbonyl in the starting material, then an isomer of glucose, fructose, is formed.

(A) Resonance structures of an enolate illustrating that there is high electron density on both the oxygen atom and one of the carbon atoms in this molecule. (B) When an enolate attacks a carbonyl group through its nucleophilic carbon, a new carbon–carbon bond is formed. If there is a good leaving group, X, on the tetrahedral intermediate species then a second carbonyl group can form, otherwise the tetrahedral species can gain a proton to form an alcohol. (C) The reaction of dihydroxyacetone phosphate (red) and glyceraldehyde-3-phosphate (blue) to form fructose-1,6-bisphosphate is a key reaction in glucose synthesis, while the reverse reaction occurs in the breakdown of glucose (glycolysis).

NAD+ is the acceptor molecule for hydride ion in many organic oxidation reactions. It also donates hydride ion in reductions of, for example, carbonyl derivatives.
Similar articles
-
Wolahan SM, Hirt D, Glenn TC. Wolahan SM, et al. In: Kobeissy FH, editor. Brain Neurotrauma: Molecular, Neuropsychological, and Rehabilitation Aspects. Boca Raton (FL): CRC Press/Taylor & Francis; 2015. Chapter 25. In: Kobeissy FH, editor. Brain Neurotrauma: Molecular, Neuropsychological, and Rehabilitation Aspects. Boca Raton (FL): CRC Press/Taylor & Francis; 2015. Chapter 25. PMID: 26269925 Free Books & Documents. Review.
-
Grimme S, Antony J, Schwabe T, Mück-Lichtenfeld C. Grimme S, et al. Org Biomol Chem. 2007 Mar 7;5(5):741-58. doi: 10.1039/b615319b. Epub 2007 Jan 26. Org Biomol Chem. 2007. PMID: 17315059 Review.
-
The polygonal model: A simple representation of biomolecules as a tool for teaching metabolism.
Bonafe CFS, Bispo JAC, de Jesus MB. Bonafe CFS, et al. Biochem Mol Biol Educ. 2018 Jan;46(1):66-75. doi: 10.1002/bmb.21093. Epub 2017 Nov 13. Biochem Mol Biol Educ. 2018. PMID: 29131491
-
Bioconjugation via azide-Staudinger ligation: an overview.
Schilling CI, Jung N, Biskup M, Schepers U, Bräse S. Schilling CI, et al. Chem Soc Rev. 2011 Sep;40(9):4840-71. doi: 10.1039/c0cs00123f. Epub 2011 Jun 17. Chem Soc Rev. 2011. PMID: 21687844 Review.
-
Reversible ligand binding reactions: Why do biochemistry students have trouble connecting the dots?
Sears DW, Thompson SE, Saxon SR. Sears DW, et al. Biochem Mol Biol Educ. 2007 Mar;35(2):105-18. doi: 10.1002/bmb.29. Biochem Mol Biol Educ. 2007. PMID: 21591070
Cited by
-
TAFPred: Torsion Angle Fluctuations Prediction from Protein Sequences.
Kabir MWU, Alawad DM, Mishra A, Hoque MT. Kabir MWU, et al. Biology (Basel). 2023 Jul 19;12(7):1020. doi: 10.3390/biology12071020. Biology (Basel). 2023. PMID: 37508449 Free PMC article.
-
Stollar EJ, Smith DP. Stollar EJ, et al. Essays Biochem. 2020 Oct 8;64(4):649-680. doi: 10.1042/EBC20190042. Essays Biochem. 2020. PMID: 32975287 Free PMC article. Review.
-
Kortel M, Mansuriya BD, Vargas Santana N, Altintas Z. Kortel M, et al. Micromachines (Basel). 2020 Sep 18;11(9):866. doi: 10.3390/mi11090866. Micromachines (Basel). 2020. PMID: 32962061 Free PMC article. Review.
-
Li Y, Liao C, Tjong SC. Li Y, et al. Nanomaterials (Basel). 2019 Jun 30;9(7):952. doi: 10.3390/nano9070952. Nanomaterials (Basel). 2019. PMID: 31261995 Free PMC article. Review.
References
-
- Davenport R.C., Bash P.A., Seaton B.A., Karplus M., Petsko G.A. and Ringe D. (1991) Structure of the triosephosphate isomerase-phosphoglycolohydroxamate complex: an analogue of the intermediate on the reaction pathway. Biochemistry 30, 5821–5826 - PubMed
Further reading
-
- Crowe Jonathan and Bradshaw Tony (2014) Chemistry for the Biosciences: The Essential Concepts, 3rd edn, OUP, ISBN-13: 978-0199662883
-
- Keeler James and Wothers Peter (2003) Why Chemical Reactions Happen, OUP, ISBN-13: 978-0199249732
Advanced level texts
-
- Keeler James and Wothers Peter (2013) Chemical Structure and Reactivity: An Integrated Approach, 2nd edn, OUP, ISBN-13: 978-0199604135
-
- Bugg Tim D.H. (2012) Introduction to Enzyme and Coenzyme Chemistry, 3rd edn, Wiley, ISBN-13: 978-1119995951
-
- Price Nicholas C., Dwek Raymond A., Ratcliffe George and Wormald Mark R. (1997) Principles and Problems in Physical Chemistry for Biochemists, 3rd edn, OUP, ISBN-13: 978-0198792819
Publication types
MeSH terms
Substances
LinkOut - more resources
Full Text Sources
Other Literature Sources